Quantum Computing: The Basics
In the realm of modern technology, one phrase is gaining considerable attention – quantum computing. But what exactly does it mean, and how is it applied in our world? In simple terms, quantum computing utilizes quantum bits, or ‘qubits’, in place of the traditional binary bits used in conventional computing.
Unlike the 1s and 0s of standard digital computers, qubits harness the unique properties of quantum physics: superposition and entanglement. Superposition allows a qubit to be in multiple states at once, while entanglement links qubits across space, creating a vast web of interconnections. These features open up new computational possibilities far beyond our traditional computers.
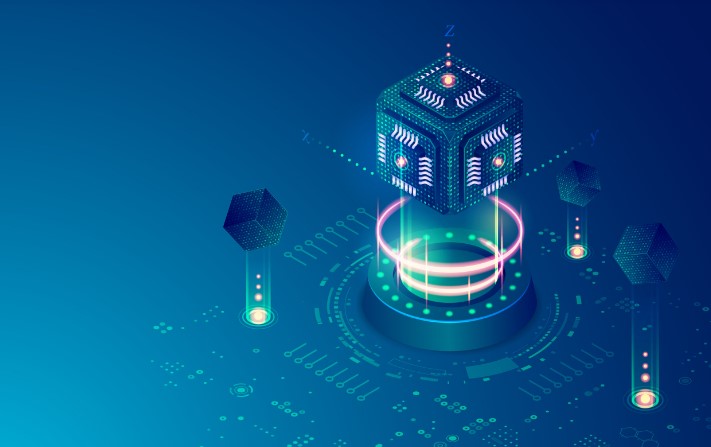
Now, as the world strides forward in the field of quantum computing, we turn our attention to the term applied quantum computing. This involves leveraging these quantum principles for solving real-world problems. It’s here, on the frontline of innovation, that quantum technology’s true potential is unfolding. In this article, we will discuss about “What is Meant by “Applied Quantum Computing?”” in details. Keep reading!
How Does Applied Quantum Computing Work?
When we speak about applied quantum computing, we refer to the use of quantum information processing to address complex challenges. It involves designing quantum algorithms to provide faster, more efficient solutions than traditional computing methods.
Quantum algorithms, like Shor’s for factoring large numbers or Grover’s for searching unsorted databases, have been proven theoretically to outperform their classical counterparts. Implementing these quantum algorithms on actual quantum hardware, however, is the grand challenge that scientists and engineers are working on currently.
Quantum Computing in Action: Practical Applications
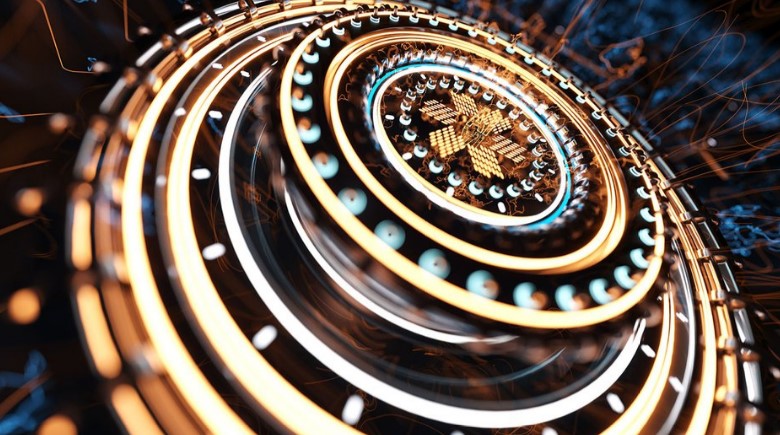
The real-world applications of quantum computing are truly breathtaking, covering sectors as diverse as cryptography, optimization, and machine learning.
Quantum cryptography, for instance, uses quantum mechanics to secure communication, providing a level of security that is theoretically unbreakable.
Quantum optimization algorithms have shown significant promise in dealing with complex optimization problems in logistics, scheduling, and machine learning.
Speaking of machine learning, quantum machine learning utilizes quantum computers to process and analyze massive data sets more efficiently than classical machine learning techniques.
Quantum Computing’s Limitations and Challenges
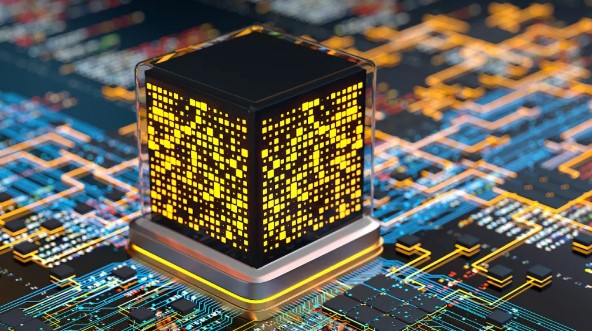
Just like any pioneering technology, applied quantum computing has its share of challenges and limitations. For instance, maintaining quantum superposition and entanglement in qubits—essential for quantum computation—is notoriously difficult. The issue of “decoherence,” where qubits lose their quantum state due to environmental interference, remains a significant challenge.
Furthermore, the technology for quantum error correction is still in its infancy. This is vital because quantum computers are inherently susceptible to errors, and reliable error correction methods are necessary for practical quantum computing.
Quantum Computing: The Future
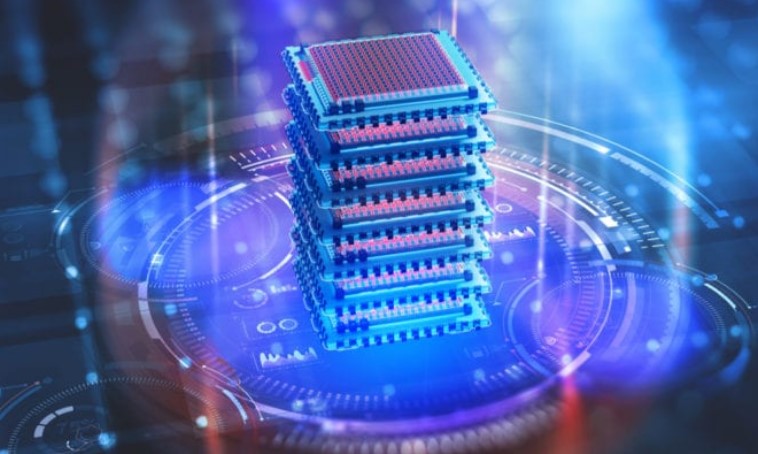
Despite these challenges, applied quantum computing holds enormous potential. Current research initiatives are consistently pushing the boundaries, with developments in quantum sensors and quantum metrology improving our measurement and detection capabilities. Quantum communication systems are also being developed that could revolutionize how we transmit information.
The question is not if, but when, these quantum technologies will reshape our world. The transition to quantum computing might seem overwhelming, but remember that every technological leap has its learning curve. And with each step forward, we get closer to a future where quantum computing is as ubiquitous as the smartphone.
Quantum Superposition and Quantum Entanglement: Twin Pillars of Quantum Computing
Underpinning the power of quantum computing are two fundamental principles of quantum mechanics: superposition and entanglement.
Quantum superposition refers to a qubit’s ability to exist in multiple states simultaneously. In traditional computing, a bit can be either in a state of 0 or 1. In stark contrast, a qubit can be both 0 and 1 at the same time, thanks to superposition. This capability exponentially increases the amount of information a quantum computer can process, unlocking the potential for incredibly complex computations.
Quantum entanglement, a phenomenon Albert Einstein famously termed as “spooky action at a distance”, is another key principle. When qubits become entangled, the state of one qubit is directly related to the state of the other, no matter how far apart they are. This linkage provides a level of interconnectedness that can dramatically accelerate the speed of quantum calculations.
Understanding and harnessing these principles are vital to the practical application of quantum computing. From solving intractable mathematical problems to modelling complex biological systems, the twin pillars of superposition and entanglement are set to redefine the computational landscape.
Quantum Gates: The Building Blocks of Quantum Circuits
Just as classical computers use logic gates to perform operations on bits, quantum computers use quantum gates to perform operations on qubits. However, unlike classical gates that perform deterministic operations, quantum gates perform probabilistic operations owing to the principles of superposition and entanglement.
This allows quantum computers to explore a vast number of possibilities at once, making them particularly effective for tasks like factoring large numbers or simulating quantum systems. The use of quantum gates to manipulate qubits is a crucial element in the development of quantum algorithms and thus, the progress of applied quantum computing.
Quantum Annealing: A Powerful Tool for Optimization Problems
Quantum annealing is a quantum computing technique used to find the global minimum for complex optimization problems. It uses the principles of quantum superposition and entanglement to traverse the solution space more efficiently than classical methods.
From optimizing financial portfolios to improving machine learning algorithms, quantum annealing can have wide-ranging applications. Quantum computers using this technique are often referred to as “quantum annealers,” with D-Wave Systems leading the way in this segment of the quantum computing market.
Quantum Metrology and Quantum Sensors: Enhancing Precision
In the world of measurement and detection, quantum technologies promise unparalleled precision. Quantum metrology uses quantum states to measure physical quantities, while quantum sensors leverage quantum phenomena to detect changes in an environment.
These technologies are expected to lead to significant advancements in various fields such as medicine, navigation, and environmental monitoring. For instance, quantum sensors could enable the detection of diseases at their earliest stages or the precise measurement of gravitational waves, opening up new frontiers in our understanding of the universe.
Also Read: 1Filmy4Wep: Downlaod Latest Movies 300mb, 720p, 480p HD Quality [2023]
Frequently Asked Questions About What is Meant by “Applied Quantum Computing?”
Q: What are quantum gates?
A: Quantum gates are the fundamental operations that quantum computers use to manipulate qubits. They differ from classical gates by performing probabilistic operations due to the principles of superposition and entanglement.
Q: What is quantum annealing?
A: Quantum annealing is a technique used in quantum computing to find optimal solutions to complex problems. It uses quantum mechanics to search the solution space more efficiently than classical methods.
Q: How can quantum metrology and quantum sensors enhance our measurement capabilities?
A: Quantum metrology uses quantum states to measure physical quantities with unprecedented precision. Quantum sensors, which leverage quantum phenomena to detect changes, can revolutionize fields such as medicine, navigation, and environmental monitoring.
Q: How do quantum computers differ from classical computers?
A: Quantum computers use qubits, which can exist in multiple states at once, unlike classical bits that can only be 0 or 1. This enables quantum computers to process vast amounts of information more efficiently.
Q: What are some real-world applications of applied quantum computing?
A: Quantum computing has potential applications in numerous sectors, including cryptography, machine learning, and optimization. For instance, quantum cryptography could enhance the security of communications, and quantum machine learning could handle complex datasets more effectively.
Q: What are the limitations of applied quantum computing?
A: Key challenges include maintaining the quantum states of qubits (known as coherence) and developing reliable quantum error correction techniques, as quantum computations are more susceptible to errors than classical ones.
Q: What does the future hold for applied quantum computing?
A: Current research initiatives in areas like quantum sensors, quantum metrology, and quantum communication are paving the way for a future where quantum computing will become a fundamental part of our technological landscape.
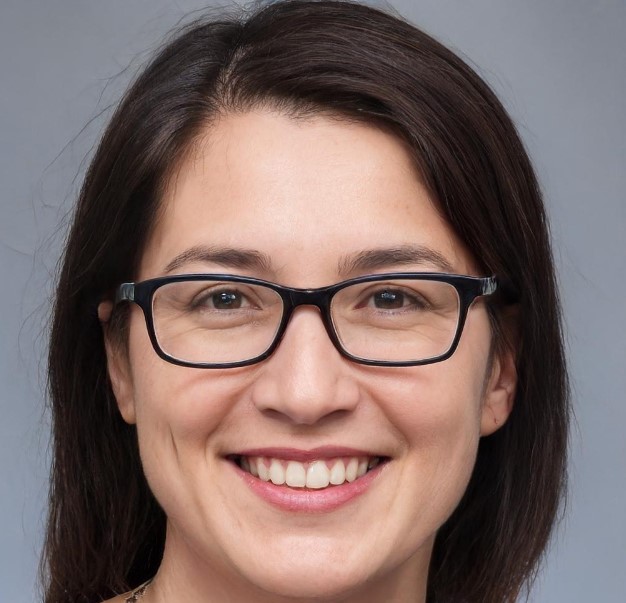
Aretha Davis, the wordsmith extraordinaire, weaves enchanting tales with her pen and keyboard. A renowned blogger and writer, her captivating prose transports readers to realms unknown. Join her literary journey and be swept away by the magic of her words.